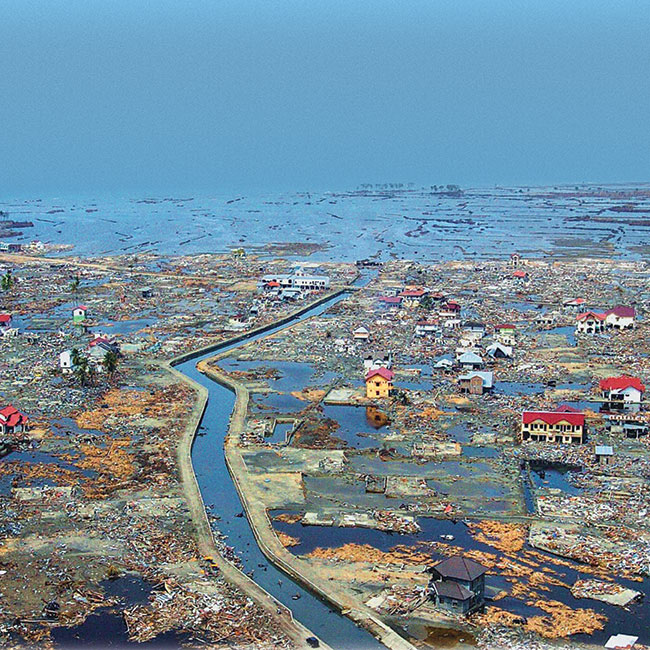
In the most basic terms, tsunamis are caused by the massive displacement of water that then ripples outward in a series of concentric waves that travel up to 500 mph from the point of origin. Tsunamis vary in size and are triggered by a variety of events, including earthquakes, underwater landslides, or volcanic eruptions. While the engineering community has spent decades improving its ability to design structures to resist the shaking of earthquakes, it has only been within the past decade or so that focus has been placed on protecting coastal communities with the development of tsunami-resistant design standards and other methods to protect people in tsunami inundation zones.
Major events have sparked this effort. The devastating December 26, 2004, Indian Ocean tsunami was generated by a moment magnitude 9.1 earthquake on the subduction zone at the interface between the India and Burma tectonic plates, where the seafloor was estimated to have been lifted by several meters. The first waves, measuring 10 to 15 m in height, arrived in northern Sumatra, an Indonesian island, within a half hour, according to the U.S. Geological Survey (USGS). The waves lessened in height the further they traveled from their origin, but Indonesia, Sri Lanka, India, Thailand, Somalia, Maldives, Malaysia, Myanmar, Tanzania, Bangladesh, and Kenya were among countries hit the hardest, according to the USGS. According to the National Oceanic and Atmospheric Administration’s National Centers for Environmental Information (NCEI) tsunami data-base, 227,899 people died as a result of the tsunami and the maximum distance that the tsunami reached inland—which occurred in Sumatra—was 5,000 m. The world was shocked.
On March 11, 2011, another devastating tsunami occurred. This one was generated by the moment magnitude 9.1 Tohoku, Japan, earthquake on the subduction zone boundary between the Pacific and North American plates. Water heights reached 20 m or higher at populated coastal prefectures in Japan, near the earthquake’s epicenter, and the first waves of the tsunami arrived approximately 25 to 30 minutes after the earthquake. The highest recorded heights occurring outside Japan—2 to 3 m—were measured in Crescent City, California, 24 hours after the earthquake, according to a special report issued by the Oakland, California-based Earthquake Engineering Research Institute in November 2011. The effects of the tsunami and its strong currents were felt in Hawaii, Chile, the Galapagos Islands, and the West Coast of the contiguous United States. According to the NCEI tsunami database, 18,454 people died and the maximum inland reach measured a whopping 7,900 m.

“The 2004 Indian Ocean tsunami really shook the world. It made people realize that tsunamis can really be life threatening, [and] it spurred the engineering community,” says Yumei Wang, P.E., F.ASCE, the resilience engineer who leads the geohazards efforts at the Oregon Department of Geology and Mineral Industries. The Tohoku earthquake gave engineers further impetus to integrate tsunami design into the building code, she says.
Within the United States, Washington and Oregon are readying for potential tsunamis due to the way that the Cascadia subduction zone, where the Juan de Fuca and North American plates meet, extends offshore in close proximity to the coastline. (The fault zone extends into the northernmost portion of California.) “It’s a type of fault system that can produce the biggest earthquakes in the world,” says Wang. “This fault stretches about seven hundred miles and can produce a magnitude nine earthquake. In the past it has produced forty-some magnitude eight or nine earthquakes, and with them come tsunamis, so you have about five minutes of shaking and about five, ten, fifteen minutes later—depending on where you are with respect to the fault system—the tsunami will hit the shores.”
Buildings and communities in this area must be ready to withstand the one-two punch of a major earthquake and a tsunami. This type of tsunami is known as a near-field tsunami, following quickly on the heels of a local earthquake. The same event could also trigger a tsunami that travels great distances across the ocean—a far-field tsunami—that strikes hours after its originating event. These latter types of tsunamis are just as capable of causing death and destruction when they reach coastlines.
The Pacific Northwest is within the “window of opportunity,” as Wang refers to it, for a magnitude 9 earthquake and subsequent near-field tsunami. On January 26, 1700, a so-called “orphan tsunami” arrived in Japan, where written records were being kept, unlike in the Pacific Northwest at that time. A modern, multidisciplinary team examined geologic evidence in the Pacific Northwest and studied Native American folklore and determined that the origin of that tsunami was a magnitude 9 rupture along the Cascadia subduction zone, according to Wang. “On average, they occur every three hundred to five hundred years. It’s been three hundred and eighteen years, and we know that we’re due for another Cascadia earthquake,” she says.

While Alaska also has an offshore subduction zone and can experience near-field tsunamis, the risk to its most populous area—Anchorage—is very low because of the natural boundaries of Cook Inlet, which protects the city, according information on Anchorage’s Office of Emergency Management webpage. Hawaii and Central and Southern California, on the other hand, do not have offshore subduction zones that can generate massive earthquakes, so their tsunami preparations focus on readying their coastal communities for the arrival of far-field tsunamis, according to Wang.
Much as they have worked to prepare the built environment for earthquakes, engineers in the United States are now doing the same for tsunamis. When it comes to protecting people, there is a crucial difference between the two, however. While the best protection strategy in an earthquake is typically to seek cover and shelter in place during the shaking—as buildings built to current codes must ensure the life safety of their occupants during an event—for tsunamis, the exact opposite is true. People in tsunami inundation zones need to head to the highest possible ground as fast as possible as soon as they’ve experienced a large earthquake, which in some cases might mean they need to climb vertically in a building.
Protecting communities in tsunami inundation zones must be twofold and include emergency preparedness and structural resilience, according to Gary Chock, S.E., D.CE, F.SEI, Dist.M.ASCE, the president of Martin & Chock in Honolulu, who wrote in response to written questions posed by Civil Engineering. Chock, the chair of the ASCE 7 Tsunami Loads and Effects Subcommittee, says that while emergency preparedness will enable the quick evacuation of people, structural resilience will make it possible for critical facilities, infrastructure, and key resources to be available for communities immediately after a disaster and during the long-term economic and social recovery. This might require local planning codes to call for critical facilities such as hospitals, schools, and first-responder structures to be moved to locations farther from the shoreline or—where possible—outside of identified tsunami inundation zones. Indeed, the Alaskan communities of Valdez and Chenega, which were decimated by a moment magnitude 9.2 earthquake and subsequent tsunami in 1964, responded by relocating to sites on higher ground with less vulnerability and exposure to future tsunamis, according to Rob Witter, Ph.D., a research geologist with the USGS’s Alaska Science Center in Anchorage who wrote in response to written questions posed by Civil Engineering. Moving entire communities is rarely practical, however.

Improving the chances of a community’s long-term functional recovery after a disaster is fundamentally about resiliency. “The largest, most damaging tsunamis are generational events, in the sense that their consequences can be felt for a very, very, very long time,” says Diego Melgar, Ph.D., an assistant professor in the Department of Earth Sciences at the University of Oregon, in Eugene. “When you think about resiliency, it’s really about how elastic a society can be. How quickly can it bounce back to some semblance of normal following one of these big crises? Insofar as we can envision what will happen with one of these big events and insomuch as we can plan for them, that means not so much that the immediate aftermath in the first few hours or days is going to be better; it means that in weeks or months or maybe even a year, we can recover some semblance of what life used to be. And that is very, very important.”
Melgar is focused on developing a GPS-based tsunami early-warning system that could work in conjunction with seismic sensors to potentially shorten the time it takes to determine a near-field tsunami’s arrival time and size—from eight to ten minutes down to one to two minutes. This is an improvement that could prove crucial to people seeking higher elevations.
The devastation wrought by a tsunami inundation can erase entire communities when those communities are unprepared. Even if a community is prepared, a certain amount of damage is unavoidable. “Resiliency is different when you think about a tsunami hazard area,” explains Cale Ash, P.E., S.E., a principal and the office director of the Seattle office of Degenkolb Engineers. “In the event of a tsunami, you may not be able to preserve as much of your physical infrastructure as we try to do when we design a high-performance building against an earthquake hazard.” This is because of the damage caused by the saltwater running through a building, potentially stripping out its interior as it carries debris inland. Debris, in this case, can range from mud, sand, and trees to boats, ships, and cars.

Still, resilient design for communities and infrastructure is the best way to withstand and recover from a disaster, according to Michael Mahoney, a senior geophysicist in the mitigation directorate at the Federal Emergency Management Agency (FEMA). “If your buildings are resilient and designed for that, instead of having to tear them down and rebuild—which is a very costly [and] time-consuming process—you can get back into [them] with minimal repairs, [and] you’re that much ahead of the curve on recovering from a disaster,” he says.
Increasingly, prepared communities will be those that ensure their new buildings in tsunami inundation zones are designed to continue safely standing as tsunamis roll through before pulling back to the sea. And these buildings can be designed to provide vertical evacuation shelters for people who might not otherwise be able to make it to safe elevations before the inundation arrives.
In support of creating tsunami-resilient structures, the most recent edition of the ASCE 7 standard Minimum Design Loads and Associated Criteria for Buildings and Other Structures, ASCE/SEI 7-16 included a new chapter (6) on tsunami design. The chapter can be used to help with planning and siting decisions, structural design, and postdisaster reconstruction, according to Chock, whose committee wrote the new chapter. The new material contains information on tsunami inundation modeling and includes technical design provisions for five different forces assessed for tsunamis: hydrostatic, hydrodynamic, debris impact, foundation scour, and site erosion.
The standard—including the new chapter—has been incorporated into the 2018 International Building Code, says Chock. It is also being adopted as part of that code by individual states. “Once this chapter is officially adopted in the five western states (with Hawaii likely later this year or early next year), all critical and high-occupancy buildings in the Tsunami Design Zone (TDZ) defined by ASCE/SEI 7-16 will be required to include tsunami-resistant design,” said Ian N. Robertson, Ph.D., P.E., S.E., M.ASCE, the Arthur N.L. Chiu Distinguished Professor in the Department of Civil and Environmental Engineering at the University of Hawaii at Manoa. Robertson, who wrote in response to questions posed by Civil Engineering, was the vice chair of the ASCE 7 Tsunami Loads and Effects Subcommittee. “In addition, we hope that many communities will also require tsunami design for taller multistory reinforced-concrete and structural-steel framed buildings in the TDZ so as to improve the number of vertical evacuation options for folks who do not make it out of the inundation zone prior to tsunami arrival,” Robertson said.

This will prove particularly important to areas such as the Pacific Northwest. “It’s obviously safer to be outside of the tsunami zone than inside of the zone,” says Wang. Oregon’s building code has prohibited the construction of certain critical facilities in identified tsunami inundation zones since 1995, she says. However, she notes: “I think that the entire Oregon coast can be transformed to a safe place from tsunamis with respect to people’s lives. And that is a very big deal, and that is the biggest step forward with ASCE 7.”
“The U.S. is now the leader in tsunami design code provisions,” said Robertson. “Although Japan has had design provisions for some years, their requirements are not comprehensive and are quite conservative, leading to more costly buildings.” In addition, the U.S. tsunami design provisions are being considered by a number of countries for possible adoption, including Chile and New Zealand, according to Robertson.
Before ASCE 7-16, FEMA released Guidelines for Design of Structures for Vertical Evacuation from Tsunamis (known as FEMA P646) in June 2008, prepared by the Redwood City, California-based Applied Technology Council (ATC). A second edition was released in 2012, and a third edition is currently in process. While the document was an important precursor of the ASCE 7-16 chapter on tsunami design, it contained tsunami loading expressions that were superseded by ASCE 7-16. The new edition is being funded by FEMA and prepared by the ATC, with Robertson acting as the lead author. It will be revised to align with the ASCE expressions and will be combined with its companion community-planning manual, volume P646A, so that all the community planning, financing, and operation of vertical evacuation structures can be found in one volume. “In addition, I added a new section on the assessment of existing buildings as possible vertical evacuation structures, since it may take some time before communities are able to finance new structures to serve this purpose,” Robertson said.

There is a potential concern over the creation of vertical evacuation structures, however, that the ATC considered when it first developed FEMA P646, according to Jon A. Heintz, P.E., M.ASCE, the executive director of the ATC. “The existence of the area of refuge might create a disincentive for the behavior that we really wanted,” he says. “The best behavior in the event of a tsunami is evacuation to higher ground.” However, he notes, in areas where near-field tsunamis can arrive quickly after an earthquake, there is no time to evacuate to higher ground and the vertical evacuation structures will be crucial to saving lives.
When designing structures in tsunami inundation zones, the consensus is that people who have evacuated will need to remain in place for about 12 to 24 hours as the waves of the tsunami ebb and flow around the structure. While the expectation is that evacuation structures will keep some portable supplies on-site, only buildings designed to operate “off-grid” will be able to collect wastewater and have freshwater and electricity available for evacuees for that long.
Vertical evacuation facilities can be incorporated into the design of critical buildings that are already being planned. Ocosta Elementary School, a $16-million project, opened in 2016 in Westport, Washington, and includes the first tsunami-resistant vertical evacuation structure built in the United States as part of a building. (Read “New Elementary School is Prepared for a Tsunami” at www.asce.org/cemagazine, under Past Web-Only News Articles.) Despite being designed before the publication of ASCE 7-16, the building meets the chapter 6 tsunami provisions because the design team worked closely with the subcommittee before the official publication of the technical requirements, according to Degenkolb’s Ash, who was the engineer of record for the school project.
The school’s new vertical evacuation structure is a 55 by 155 ft building that also houses the gym, cafeteria, and music room. Tsunami inundation modeling—which defines the key flow and velocity characteristics of the tsunami design—showed that the safe refuge needed to be 25 ft above grade, according to Ash. The refuge has been sized for 1,000 people with 8,500 sq ft of space, but the structure was designed with the same assembly load capacity as a concert hall or exit stairs so that it could be used by as many people as could fit, according to Ash.
The gymnasium was “hardened” for the arrival of a tsunami with reinforced-concrete stair towers with 14 in. thick concrete walls located in each of the building’s four corners. “Those provide the lateral stability for the building and also serve to support the gravity loads for the roof,” Ash notes. Ten steel columns that have been encased with concrete up to the site’s expected 14 ft inundation depth surround the perimeter of the building. “We tried to have as few vertical elements as possible because anything that supports the roof structure is also vulnerable to debris impact,” Ash says. “And we designed the roof structure such that if any one of those columns on either side of the building were to be damaged due to debris impact, the roof framing could just redistribute the forces and prevent progressive collapse.”
The building sits atop a 50 ft deep foundation that uses 24 in. diameter, reinforced, auger-cast piles, with additional piles located under the building’s perimeter steel columns.
Another example of tsunami-resistant design is the Oregon State University Marine Studies Initiative (MSI) building, located at the Hatfield Marine Science Center in Newport, Oregon, which broke ground this year. This approximately $45-million project will combine internal and external vertical evacuation structures so anyone nearby can seek safety. As in the state of Washington, vertical evacuation structures within the tsunami inundation zone in Oregon must be ready to operate safely despite the expected five minutes or so of shaking that a moment magnitude 9 earthquake would generate.
While the MSI appears visually as one building, it performs structurally as two separate buildings designated as the lab and the commons. The lab is a three-story building with three reinforced-concrete cores that have been founded atop two large mat footings, according to Seth Thomas, P.E., S.E., M.ASCE, a structural engineer at KPFF in Portland, Oregon, and the firm’s project manager for the MSI. The cores extend above the roofline to create small penthouse structures and provide access to the rooftop evacuation area that has a capacity for at least 900 people. The lab is rectangular in plan and features steel gravity framing with a concrete-on-metal-deck floor system. The roof is a structural slab, according to Thomas. The steel frame has been designed to resist progressive collapse.
The commons is a similar two-story structure, and much of the internal space is two stories in height, according to Thomas. This building supports an external vertical evacuation ramp, which extends from the ground to the roof of the lab. This “intuitive vertical evacuation” system will make it easy for even those unfamiliar with the area to get out of the way of the tsunami, he says.
Both wings are designed to perform safely during the maximum considered earthquake, but only the lab is designed to resist the maximum considered tsunami. The lab was designed for impact loads from debris, including loads that might be imposed by a 100 ft long ship, the largest that typically dock at a nearby marina. The nonstructural elements of the lab’s first two floors have been designed to wash away with a tsunami.
Extensive soil improvements will be made and foundations built to support the lab. “The building is like an iceberg in that a significant amount of the structure is actually underground,” said Thomas Robbins, AIA, LEED BD+C, a principal of Yost Grube Hall Architecture, who wrote in response to questions posed by Civil Engineering. The soil conditions at the site consist of dredged sand fill and alluvial sand to depths of about 90 ft, under which is about 20 ft of silt overlying soft rock—specifically, siltstone, according to Jason Bock, P.E., M.ASCE, an associate at the geotechnical engineering company GRI, in Beaverton, Oregon, and the project manager for his firm on the MSI building. Bock also wrote in response to questions posed by Civil Engineering. “The largest contribution to the seismic hazard at the site is due to the Cascadia subduction zone, which extends under the site at depths of 20 to 30 km,” he explained. “During a code-based event, we estimate the unmitigated saturated sands will liquefy and experience significant postearthquake reconsolidation settlements.” These sands could also see significant scouring during a design-level tsunami, he says.
The site’s liquefaction and scour potential will be jointly mitigated by the installation of 100 ft deep soil-mixing columns that will be installed in an overlapping grid formation. This method involves mixing grout into the sand to create something similar to low-strength concrete, Thomas says. “The design criteria called for…a very low-strength concrete, but when it comes to resisting scour, it’s more than effective,” Thomas notes. In addition, hold-down anchor rods that extend 30 to 50 ft into the improved soil columns will resist buoyancy forces during a tsunami. “We looked at all kinds of different foundation systems, and the deep soil-mixing option selected provided the best bang for the buck for solving a lot of different problems,” he notes.
“Anything is doable, but the real teaching moment is how you can achieve such a project within a reasonable budget,” Robbins added.
Buildings that incorporate vertical evacuation into their structures are not people’s only protection from tsunamis. Another option, of course, is purpose-built structures. The Shoalwater Bay Tribe is building a freestanding, 40 by 50 ft in-plan, steel-frame tower that will accommodate 400 people once it opens in Tokeland, Washington. The $3.2-million, two-story evacuation structure is still being designed and will be an openplan space with two evacuation levels accessed from the ground by stairs. Currently, it is expected to be supported by six columns that will be founded on deep piles. Construction of the tower is anticipated to begin by the end of 2019.
Given the space, time, and money, a larger locality could create a community-wide master plan that would provide vertical evacuation structures dotted around a community while locating critical buildings outside the inundation zone altogether. This is already happening in Seaside, Oregon, according to Wang. The town sits in a flat, coastal area with two rivers that extend through it, parallel to the coastline, before emptying into an oceanside estuary. “Engineers should be working with the community in multidisciplinary ways to come up with community-wide solutions,” Wang says. “So, for the city of Seaside, for example, it’s not just building one tsunami evacuation structure where there is a new hotel going up, it’s really [asking] ‘How do we make sure that there are strategically placed tsunami evacuation structures throughout the whole community so that the entire community can be served?'”
The same community has also broken ground on a new school campus for kindergarten through high school that is located above the tsunami zone. When and if the time comes, it can serve as a center for community recovery efforts, she says.
It is also possible that existing buildings could function as vertical evacuation structures. To this end, Ash is working with Pasadena, California-based Tetra Tech Inc. to examine the Honolulu and Waikiki areas of Hawaii, which are located close to the ocean and need to be prepared for far-field tsunamis. “A Cascadia or Alaska or Japanese earthquake could generate a tsunami that would impact the Hawaiian Islands,” Ash explains. “And on Oahu, they may not have enough time [to evacuate]—even if it’s four to six hours of warning—because there are just so many people and the traffic is so bad that they might not be able to evacuate everyone horizontally.” The project aims to develop a procedure for the city and county of Honolulu to use in evaluating existing buildings for vertical evacuation potential, much as Seismic Evaluation and Retrofit of Existing Buildings, ASCE/SEI 41 does for earthquake performance, according to Ash. The new ASCE 7-16 chapter 6 will be integral to this effort. “A lot of it points back to the chapter six provisions, just interpreting them through the lens of an existing building,” Ash says. “An existing building is not going to have everything you want when it comes to a vertical evacuation structure, but what are the things that we think are more important to screen and evaluate for to identify viable buildings before completing a detailed evaluation?”
Of course, there are challenges with helping communities plan for disasters, especially those that are only now being identified as requiring serious planning. “It’s really a question of awareness and getting people to recognize the hazard enough to make the investment,” Mahoney says.
“Every engineer involved in planning and designing a building against tsunami effects must be able to take on the role of the protector of the people that must be saved in that moment of the impending tsunami,” said Chock.
Engineers also have a responsibility to encourage building owners and developers to look at the big picture, according to Robertson. “We will need to persuade them that it is beneficial from a life-safety and community-resilience perspective to include tsunami design for regular multistory hotels, condominiums, office buildings, et cetera, located in the tsunami design zone,” Robertson said. “In fact, I see numerous opportunities for private developers of these types of buildings to cover the cost of tsunami design through community concessions such as increased density, building height limits, et cetera. For example, I could imagine a coastal community offering a waiver of current building height limitations for a hotel developer if they were to design the building as a vertical evacuation structure for tsunamis and allow the public to use the roof as the evacuation zone during future warnings.”
Such a trade-off could be particularly important in some communities, according to Chock. “In some communities the zoning code height restrictions would mean that buildings tall enough to be safe cannot be built. That would mean the near total devastation of the population,” he explained.
The financial burden of designing for tsunamis in areas with high earthquake hazards is not onerous, according to Robertson. “We are finding that for prototypical seven-story reinforced-concrete buildings that are designed for the high seismic conditions typical of the United States’ Pacific coast, the additional cost to include tsunami design will be well less than about five percent of the building cost,” Robertson pointed out.
Of course, the argument could be made that devastating tsunamis of this size might not arrive during the expected design life of a particular building. “We will need to show that the benefits to the community of peace of mind justify the added cost even if there is never a damaging tsunami,” argued Robertson. “For example, I estimate that over the life of the Ocosta school’s vertical evacuation building, the cost to the community is less than twenty-five cents per student per day. This buys peace of mind for all of the parents of students at the elementary and middle/high schools that their children will be safe during future tsunami warnings and they do not have to rush into the evacuation zone to try and save their children.”
To learn more about tsunami modeling and the updates available in ASCE 7-16, plan to attend the Structures Congress 2019, which is hosted by ASCE and its Structural Engineering Institute (SEI) and will be held April 24-27 in Orlando, Florida. For more information and the full technical program, visit www.structurescongress.org .
Catherine A. Cardno, Ph.D., is the senior editor of Civil Engineering.
Fonte ASCE